Ready to proof
CB proofed 7/11 - left comments for Niki
Revised Niki 7/12/23
KJ proofed on 7/12 - I left one note for Niki below one of the tables.
Credit: Andrei Nasonov, Creatas Video+ / Getty Images Plus, via Getty Images.
On Mechanical Properties, Water Absorption, and Adhesion of Acrylic Elastomeric Waterproofing Coatings
The Effect of Microfibrillated Cellulose
By Otto Soidinsalo, Harrison Christopher Gallantree-Smith, and Scott Mouw, Borregaard AS, Sarpsborg, Norway
The primary function of acrylic roof coatings is to protect the underlying material from water, UV radiation, chemicals, and wear. They are typically applied either by roller or airless spray, from which the latter is increasing its share as the formulation technology is developing. Acrylic elastomeric coatings differ from standard architectural paints, as they typically have higher binder levels, as well as higher solids and have a typical viscosity around 100-130 KU.
Typical requirements for elastomeric roof coatings are high tensile strength with good elasticity, as well as good reflectivity and UV resistance. As roof coatings are exposed often to ponded water, good water resistance is also essential. However, some breathability is often needed as most of the roofing materials, such as concrete and tiles, allow the moisture to transfer from the inside of the building. Naturally, the need for breathability depends on where the building is located. Water-vapor drive is generally from areas of high vapor concentration to low vapor concentration, or from high to low temperature, meaning that the breathability is often more relevant for cold environments. In addition to good film properties, rheological properties are also important. In addition, elastomeric roof coatings are applied on several different materials, including new and aged ones, meaning that good dry and wet adhesion on various materials is essential.
General challenges related to the formulation of elastomeric roof coatings with traditional thickeners are related to water sensitivity, applicability, flow and levelling, sprayability and sagging, storage stability, as well as mud cracking. For example, cellulose ethers easily absorb water, which leads to reduced water resistance. They often suffer from poor levelling and flow, which will narrow down the available application methods. On the other hand, associative thickeners behave like surfactants, increasing the water sensitivity. They are also sensitive to co-solvents and surfactants, leading to reduced formulation stability. Both cellulose ethers and associative thickeners are known to negatively affect skinning, causing mud cracking of the film.
The compatibility and rheological behavior of microfibrillated cellulose (MFC) has previously been studied in water-based acrylic and epoxy systems.1,2 The results from these studies showed that MFC is dispersed effectively into the resins and showed good compatibility. In addition, the viscosity and sag resistance increased with increasing concentration of MFC. Furthermore, the dispersions were heat stable in terms of sedimentation and retention of rheological properties.
Microfibrillated Cellulose
Microfibrillated cellulose was first invented back in the 1980s.3 Since then, its potential and possible functionalities have been reported extensively, ranging from being used as a thickener in cosmetics to use as an oxygen barrier in food packaging.4 Until the beginning of 2010, the research and small-scale production of MFC was conducted mainly at universities and small pilot facilities. Borregaard AS launched the commercial production of MFC, opening the world’s first 1,000 dry MT Exilva factory in 2016.5
MFC consists of long and thin cellulose fibrils that form a three-dimensional (3D) network (Figure 1).6 The secret behind the unique properties is the extremely large surface area, combined with numerous hydroxyl groups on the surface of the fibrils. The interaction of MFC is based on physical as well as chemical interactions within the formulation. MFC has a strong impact on the low-shear area of the formulation and possesses significant impact on yield stress, and is shear thinning and has a high water-holding capacity. The insoluble nature of the cellulose fibrils also leads to excellent stability and compatibility. MFC is stable at a wide pH range (1-13) and is compatible with common water miscible organic solvents as well as with surfactants.
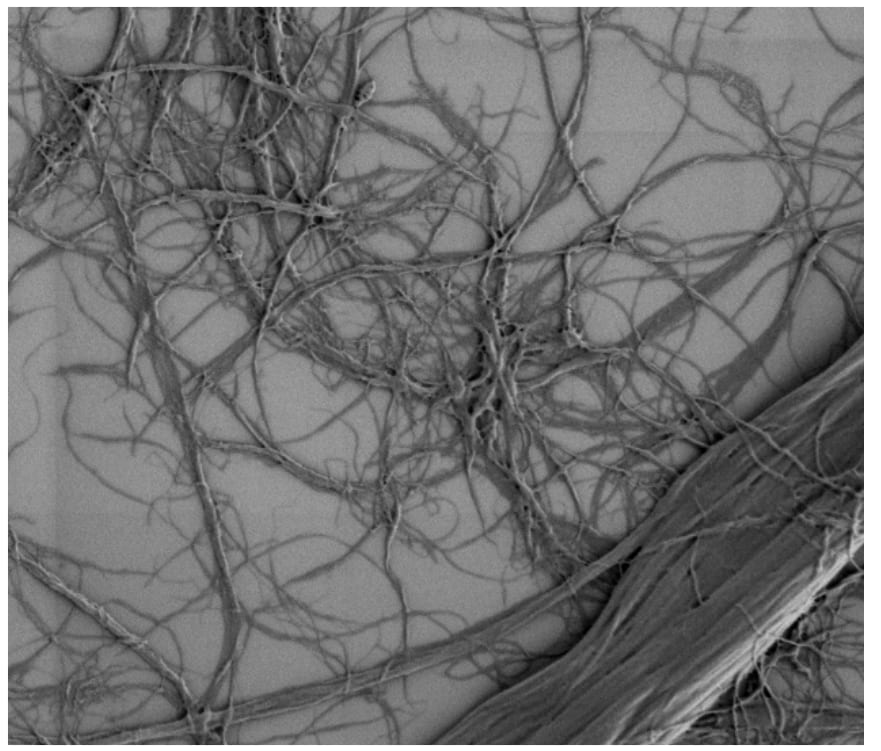
FIGURE 1 ǀ Scanning electron microscopic (SEM) picture of MFC.
Compared to common polymer solutions in water, the rheological properties of MFC suspensions are quite different. The impact of MFC on a mid-shear area is low and there is basically no impact on the high-shear area. MFC has a strong impact on low-shear and no-shear areas (Figure 2) and shows 100 times higher viscosity compared to a highly viscous HEC in low-shear areas. This leads to significantly better sag resistance, as well as storage stability. On the other hand, the impact on the KU area is significantly lower. This needs to be kept in mind as the evaluation of the impact of MFC to the rheology of the formulation can be challenging. When traditional methods, such as Brookfield or Krebs viscometers, are used, the impact of MFC cannot be seen due to the extreme shear thinning. Instead, either a rheometer or more practical methods such as sag or settling testing needs to be applied.
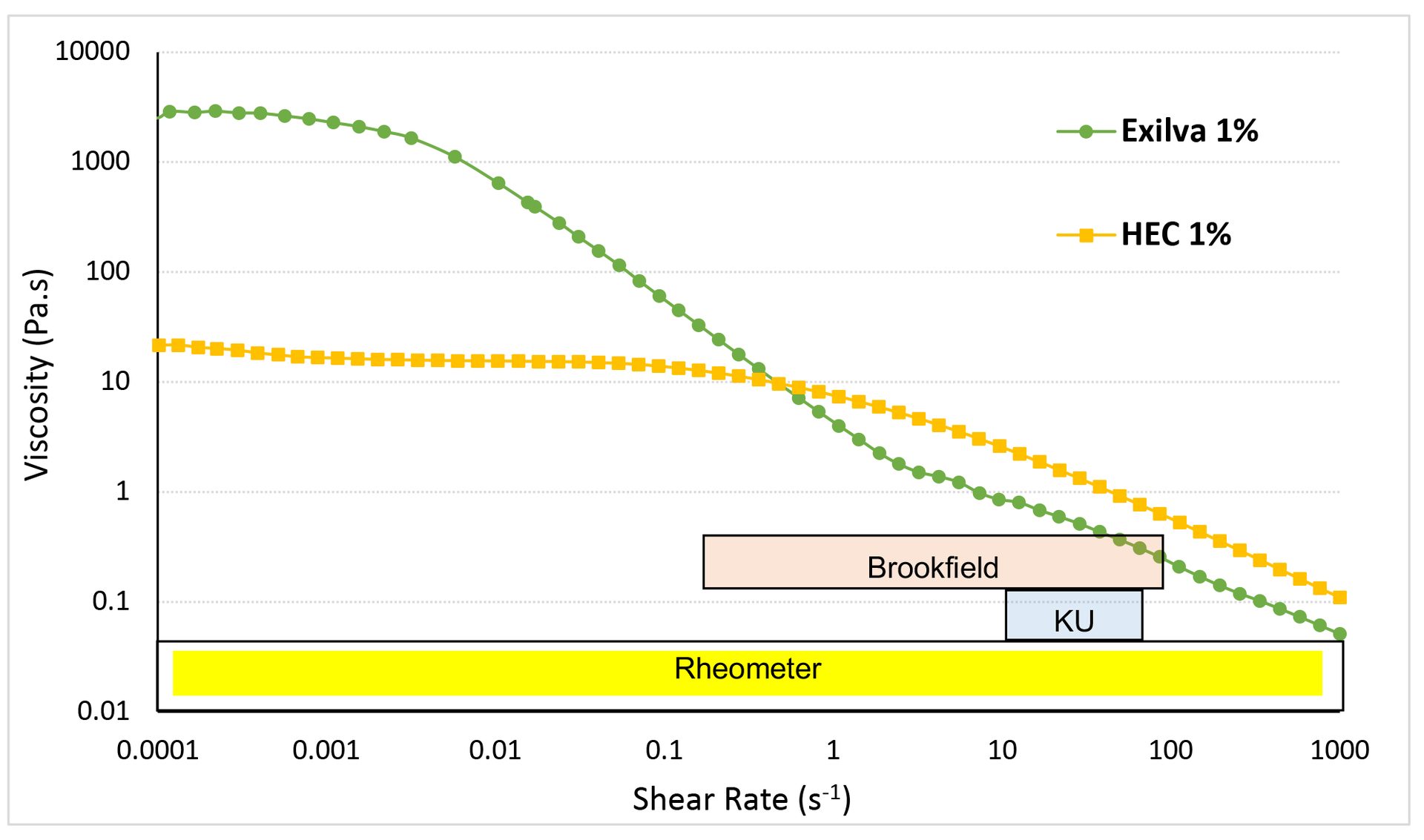
FIGURE 2 ǀ Flow curves for 1.0% (wt.%) of MFC (dot) and HEC (square).
Due to the high viscosity at low-shear rate and shear-thinning behavior, MFC can also be used as a thickener in products that are intended to be applied by spraying. MFC secures high stability during storage and allows for the spraying of very thick suspension since the viscosity decreases during the spraying. However, when the product has been applied and no forces are present, the viscosity recovers rather quickly, and the product remains where it impacted the surface, without sagging. In addition to anti-sagging, the use of MFC leads to a non-dripping effect, which is often desired in coatings.
Materials and Methods
Commercially available MFC was used in this study (Exilva® F 01-V) containing 10 wt% microfibrillated cellulose in water (manufactured by Borregaard AS). Sag resistance was determined according to ASTM D4440, adhesion according to ASTM D4541, tensile strength according to ASTM D2370, elongation according to ASTM D2370, water absorption according to an in-house method, and water vapor permeability according to ASTM D1653.
Results and Discussions
In order to understand the effect of MFC on the mechanical and rheological properties of waterproofing elastomeric coatings, a standard acrylic elastomeric roof coating formulation was chosen as a model formulation (Table 1).
Two different rheology modifiers were studied, hydroxyethyl cellulose (HEC) and MFC as a functional additive. In all cases, HEC was used to adjust the mid-shear (KU) and high-shear viscosity (ICI).
TABLE 1 ǀ Acrylic elastomeric roof coating formulation.
*As delivered
All the formulations had comparable KU viscosities due to addition of HEC to the formulations, based on MFC. The impact of MFC to the sag resistance can be seen in Table 2. As the dosage of MFC increases, the sag resistance is increased from 18 wet mils to 25 wet mils on a sealed chart.
TABLE 2 ǀ Acrylic elastomeric roof coating formulation test results.
The formulations were further evaluated in terms of film properties. The differences in the mechanical properties of the formulations are shown in Figure 3. Tensile strengths of the coatings are increasing as the dosage of MFC is increasing. This is due to the hydrogen bonding of the strong fibrils with the binder, leading to a strong but flexible network. The tensile strength of single-cellulose fibrils ranges typically between 1.6-3 GPa.7 The microfibrils in MFC consist of both crystalline as well as amorphous regions. Under stress, the amorphous regions offer flexibility due to the lower modulus.8 The impact of MFC to the elongation is noticeable when compared to the reference based on HEC, but as the dosage of MFC is increasing, the change in elongation is reduced.
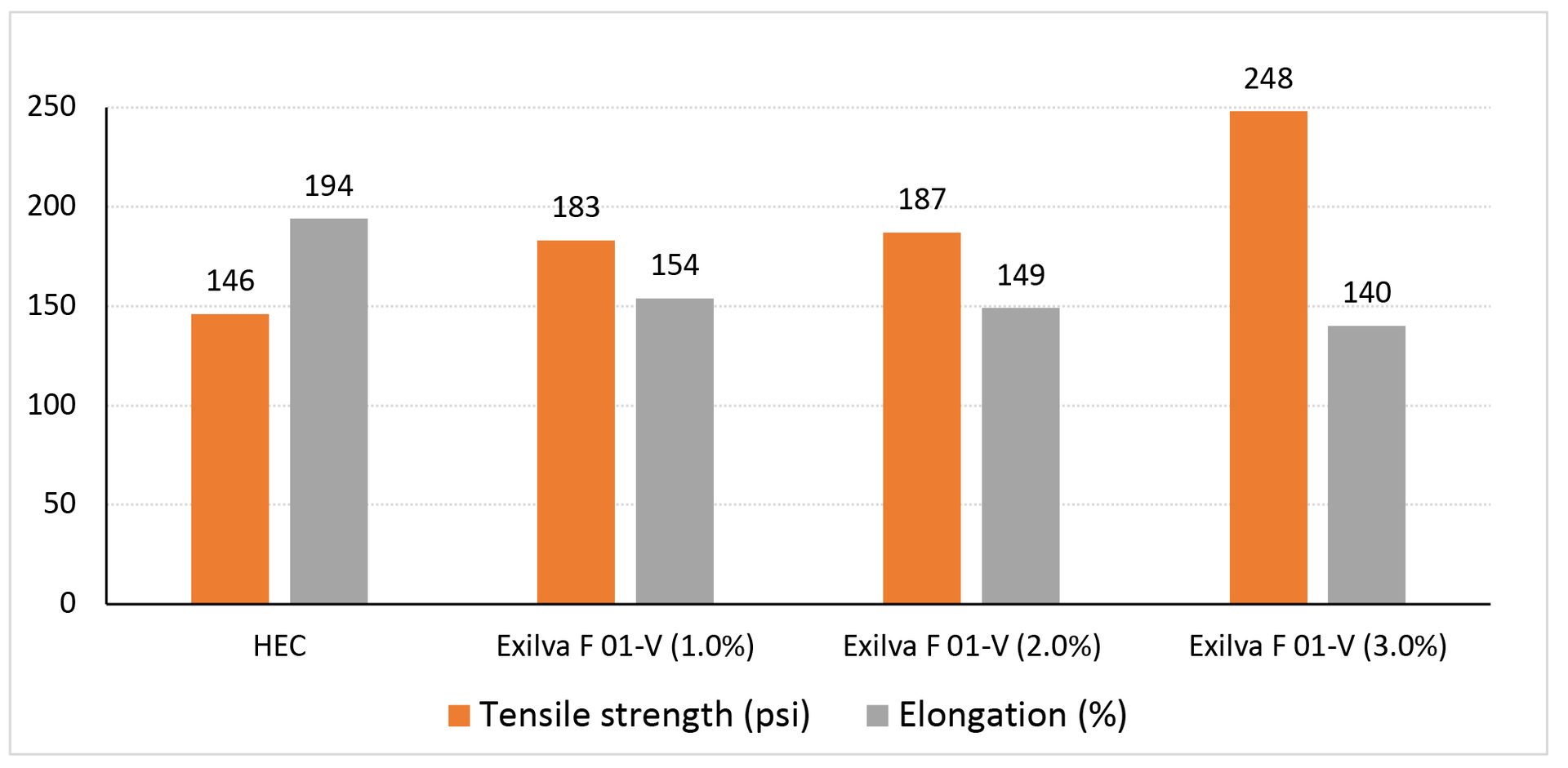
FIGURE 3 ǀ Acrylic elastomeric roof coating formulation test results.
In addition to the increase in strength, MFC reduces the water absorption of the elastomeric coatings. The level of water absorption of the coating depends typically on the thickener used, but naturally the binder also plays a role. In this particular case, the water absorption of the coatings is reduced by nearly 70% (35.4% with HEC vs 12% with MFC) with the highest MFC dosage. This is explained by the strengthening effect of the fibrils, which prevent the swelling of the film. However, it is interesting to note the dramatic impact with a low dosage of MFC (MFC 1). There were no noticeable differences in the water vapor permeability or water ponding of the coatings.
TABLE 3 ǀ The impact of MFC on the permeability of the acrylic elastomeric roof coating formulation.
The impact of MFC can be seen on the adhesion of the coating on concrete and metal surfaces in Figure 4. The adhesion (psi) on concrete increases once MFC is added but it does not increase as the dosage is increased. The impact is probably related to the higher water holding of the coating, resulting in better film formation.
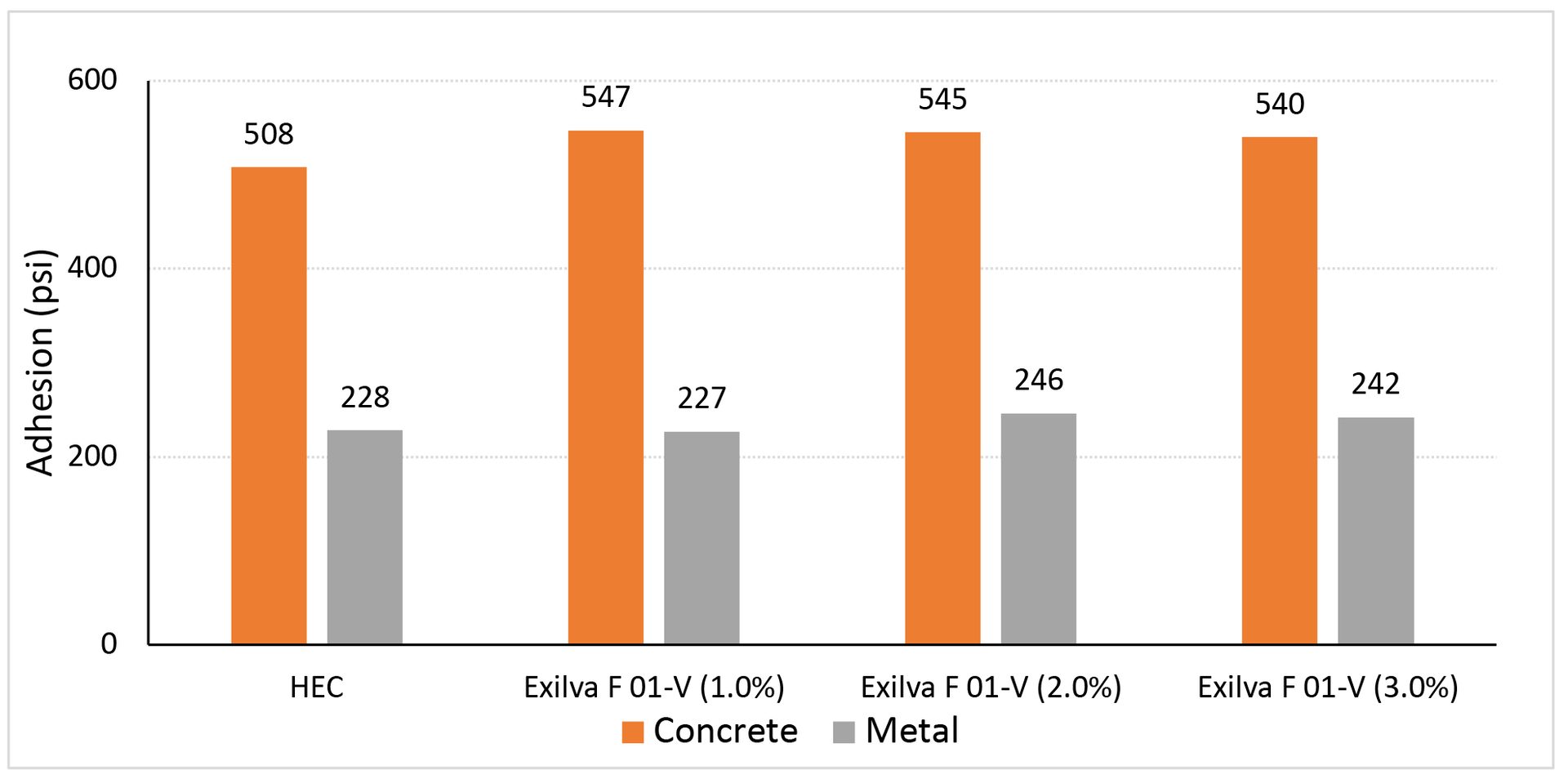
FIGURE 4 ǀ Acrylic elastomeric roof coating formulation test result.
Conclusions
The effect of MFC on rheology of the elastomeric roof coating formulation, as well as film properties, is prominent. The effect of MFC is especially significant on the mechanical properties of the acrylic elastomeric roof coating, as well as on water absorption of the coating. Tensile strength of the coating is up to 70% higher, when compared to traditional technologies, without significantly reducing the elasticity of the coating. The water absorption of the coating is reduced by nearly 70% compared to the formulation based only on HEC. Addition of MFC into elastomeric waterproofing coatings allows formulators to develop paints with higher performance, and better applicability and durability.