Ready to proof -- Clare 05/6/22
KJ proofed on 5/16 and sent corrections to Clare
Revised on 5/16
Ready for author
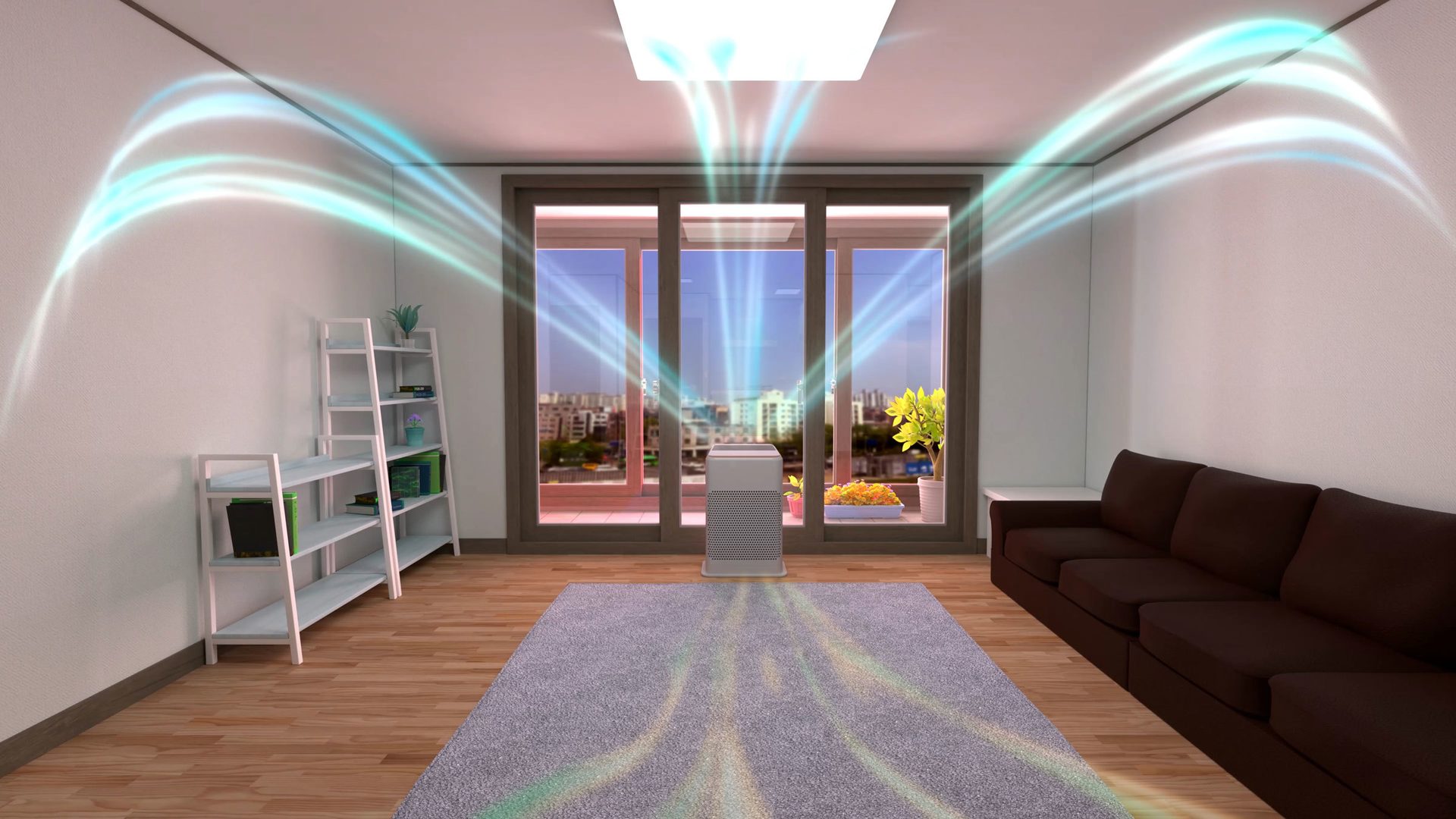
Photo: JUNHO JI, iStock/Getty Images Plus, via Getty Images
Photocatalytics
in Coatings for VOC Reduction
By John Schierlmann, Director of Research and Development, Bona Corporation, Monroe, NC
Indoor air quality is becoming a growing concern for consumers and business owners. Indoor air pollutants (VOCs — volatile organic compounds) can come from sources such as carpets, furnaces, furniture, insulation, pets, refuse and fuels from the garage (Figure 1). While current technology in home building makes houses with fewer air turns and that are far better insulated, this can trap VOCs within a house, and lead to increased asthma and allergies for the occupants.
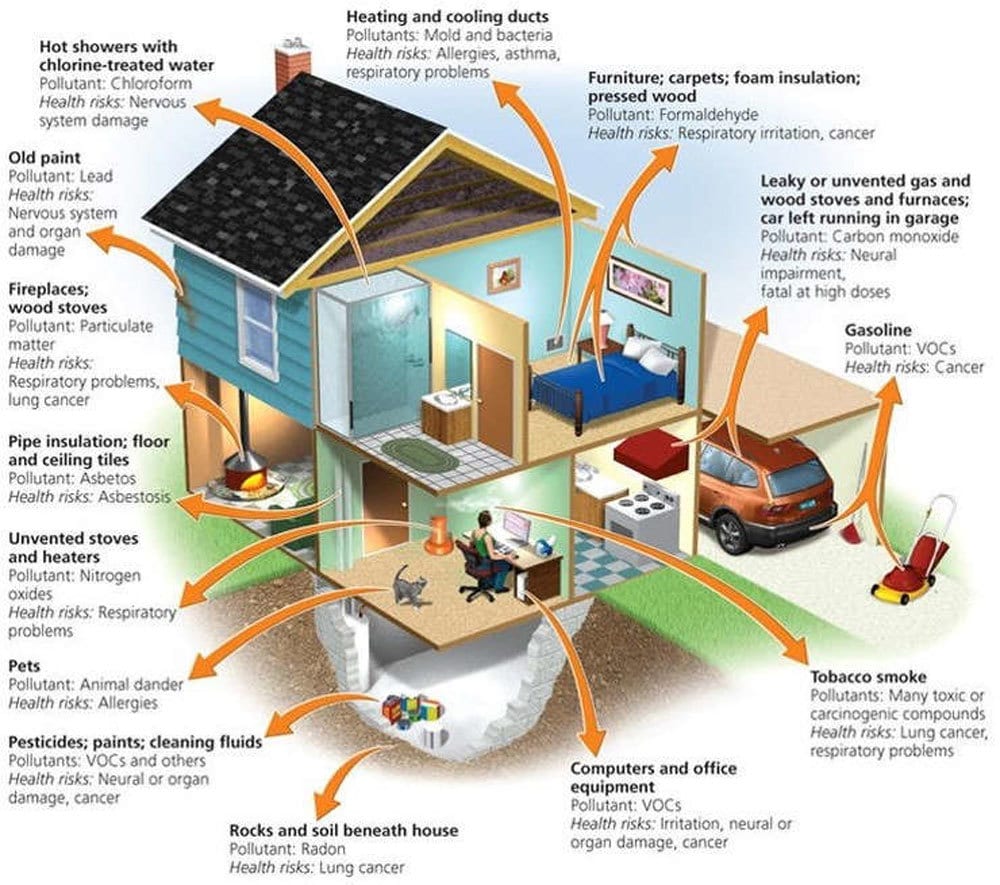
FIGURE 1 ǀ Sources of indoor air pollutants (VOCs).
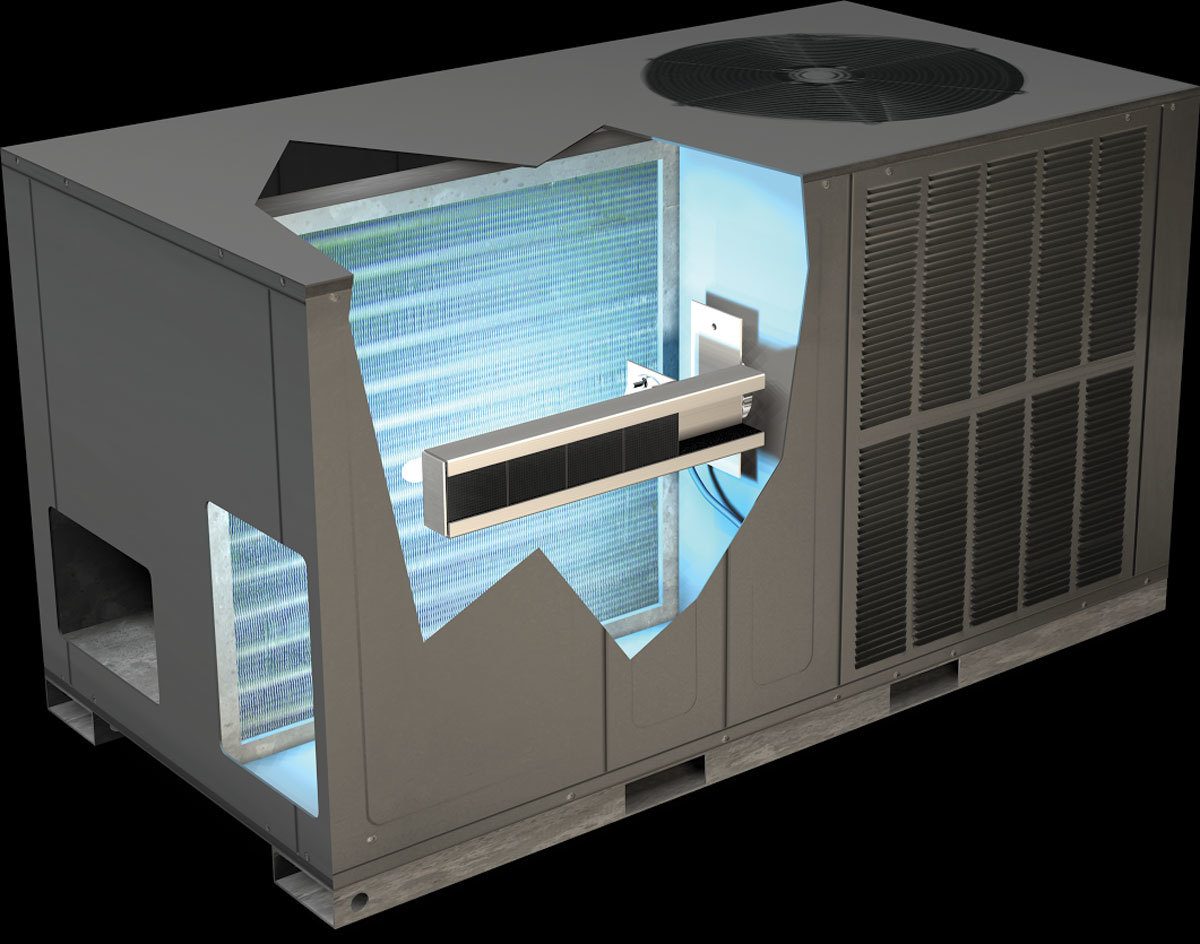
FIGURE 2 ǀ Photocatalytic HVAC system.
Products are available that claim to clean VOCs from the indoor air. These is not a magic panacea to solve all issues. The hybrid light/filter systems are costly and cover a small area for efficiency. There are also hybrid systems that are modifications to HVAC systems that are efficient but costly. Other machine types can create ozone, which poses other health concerns. Some that contain filters that can become inactive as they fill with impurities and lose their VOC reduction efficacy.
Hybrid systems such as the Molecule or HVAC systems (Figure 2) with UV lights to degrade indoor toxins, and in some cases bacteria, share a technology called photocatalytic oxidation as the active component to cause a chemical degradation.
There are several metal oxides, such as titanium dioxide, zinc oxide and copper oxide, that are utilized in the process of photocatalysis. These can be applied via chemical treatment in a high-temperature chemical “bath” and fused to the surface of more inert and stable structures such as diatoms. The metal oxides are 10-20 nanometers (Figure 3), whereas the diatoms 10-50 microns (Figure 4) are far larger in particle size, leading to a very stable and durable material.
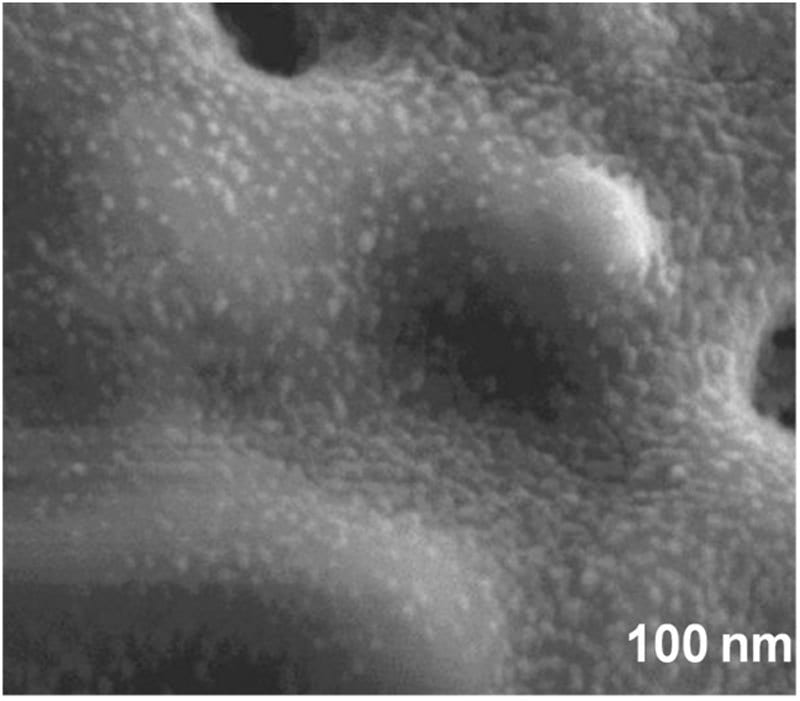
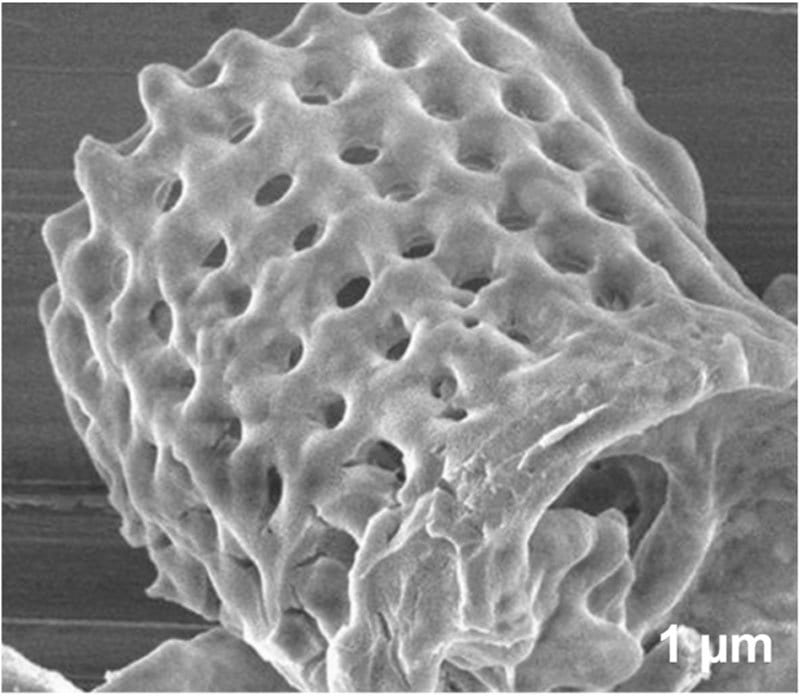
FIGURE 3 ǀ Titanium dioxide 10-20 nanometers.
FIGURE 4 ǀ Diatom 10-50 microns.
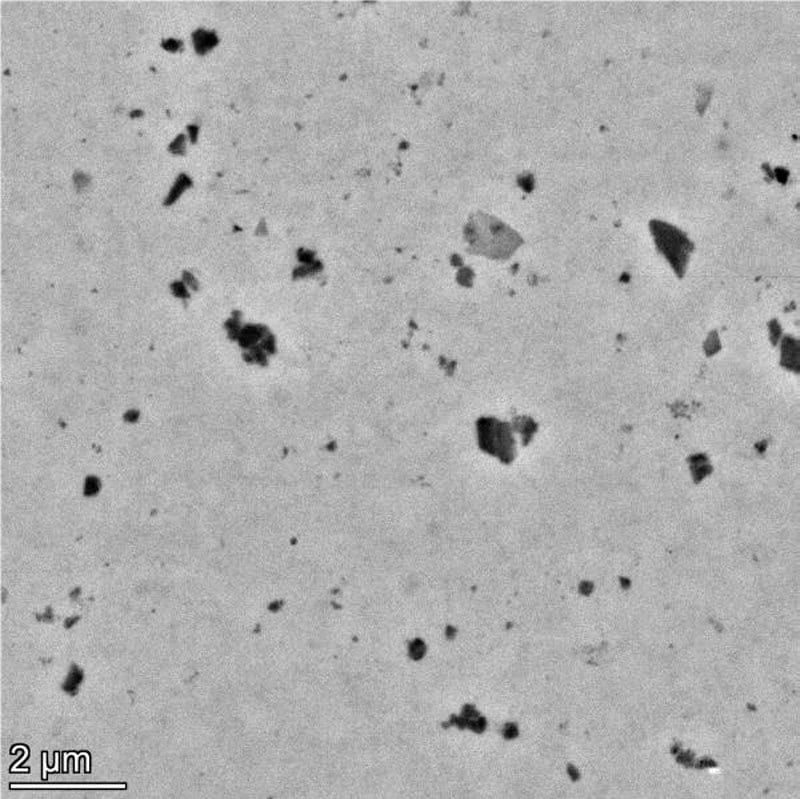
FIGURE 5 ǀ Diatom particles in polish utilizing TEM.
This material can be either added directly into the coating, if lower in spectral gloss <50 @ 60 degrees, or a dispersion can be made with a smaller particle size 0.5-3 microns. This can be added to higher gloss systems without affecting gloss level or haziness. To illustrate how the pigments dispersed into the coating, we had a third-party lab run TEM (transmission electron microscopy) images (Figure 5).
The mechanism of degradation is UV, LED or visible light striking the titanium catalyst on the diatom. The energy most efficient to accomplish this is around the 400 nanometer wave length. The diatom, with the nanoparticle titanium dioxide dispersed in the coating (as illustrated in Figure 5) causes an electron to jump energy states. This free electron reacts with the VOC, breaking the molecule into smaller species (Figure 6). The degradation process illustrated is that of formaldehyde CH2O — an oxidation/reduction to H2O and CO2.
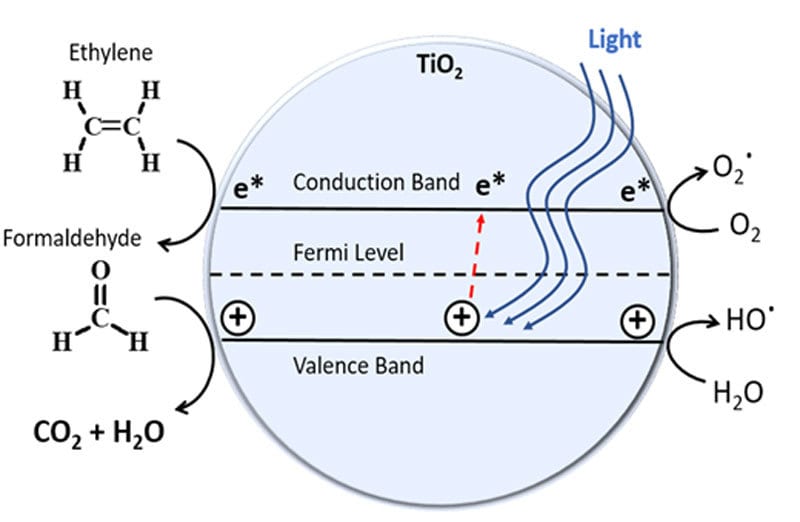
FIGURE 6 ǀ Photocatalytic degradation mechanism.
TABLE 1 ǀ Bond energies.
Obviously, all bond energies are different. Some require more energy than others to break the bonds. The energy band gap for the titanium dioxide is around 3 electron volts, which is enough to disrupt all but a carbon oxygen double bond. Table 1 illustrates some of the energy required to disrupt typical bonds.
To validate how effective the titanium dioxide was, we put together several coatings systems but will only discuss three. These all used the dispersion form that was added to the coating systems. The coatings we tested were a low-solids gloss polish, a higher solids gloss polish and a satin 2K urethane. All three were water-based.
To monitor and track this degradation process we utilized the ISO 22197-4 standard for formaldehyde reduction and ISO 22197-5 for methyl mercaptan reduction. This was conducted in test chambers at RTP labs in Raleigh, NC. RTP labs utilized a 27-liter chamber for tighter experimental control. Testing was conducted on glass panels 7x14 inches, to not influence testing. One gram of coating was weighed and applied to the glass plates and allowed to cure for 30 minutes at 25 °C and 40% relative humidity. A control, with none of the active titanium dioxide, was run in one set of tests, and an active sample with a specific amount of titanium dioxide was run. The chamber was sparged with nitrogen to eliminate any VOC in the chamber.
In the test run with gloss low-solids polish, 5 parts per million of formaldehyde was added at time zero and monitored up to 72 hours. In another test run, 2.5 parts per million methyl mercaptan was added at time zero and monitored up to 72 hours. Outside the chamber, T8 LED lights were utilized as the energy source and left on the entire time of testing.
The testing was conducted on two polishes, and a catalyzed 2K water-based urethane. The first test was conducted on a low-solids (10% non-volatile material) content gloss polish for retail consumer applications. The loading level of the titanium dioxide was 0.15% dry active in the polish (Table 2). The reduction of formaldehyde was a little lower, 13% over the control for the low-solids polish likely due to loading level, but more efficient on methyl mercaptan with a 39% reduction compared to the control.
TABLE 2 ǀ Test 18-009 gloss polish.
The amount of formaldehyde and methyl mercaptan were increased with a higher solids (22% non-volatile material), buffable Jan/San type polish (Table 3). The loading level of the titanium dioxide was 0.5% dry active in the polish. The reduction of formaldehyde was more pronounced, 93% reduction over the control. The reduction of methyl mercaptan was 36% over the control.
TABLE 3 ǀ Test 18-045 high-solids polish.
The amount of the formaldehyde in the test was 25 parts per million, and methyl mercaptan was not tested for this product (Table 4). The loading level of the titanium dioxide was 1% dry active in the polish. The reduction of the formaldehyde versus the control was 82%.
TABLE 4 ǀ Test 21-047 2K urethane.
The testing consistently showed a better reduction of the formaldehyde and methyl mercaptan (in the case of the polishes) than did the natural degradation of the formaldehyde in the control. There is also some natural degradation of the formaldehyde and methyl mercaptan over time. The reduction values were compared to the final control degradation.
Conclusion
While we were able to prove reduction of formaldehyde and methyl mercaptan in a controlled chamber, it is more challenging to demonstrate this in a room in a house. There are several factors affecting this, such as the air turns in a house, the level of insulation, and the level and source of the VOCs being emitted. We will be spending more time investigating in controlled rooms in houses.
The author would like to give a special thanks to Pietrina Peschel, Senior Chemist, Bona Corporation; Nicholas Day, Ph.D. (and team), CEO, Diatomix Corporation, (supply of photocatalytic titanium dioxide and background materials); and Alston Sykes, Principal Chemist, RTP Laboratories Inc. (analytical testing and VOC emissions).